Let’s start at the beginning, in other words, the what: the construction of the International Thermonuclear Experimental Reactor (ITER). In other words, after the Manhattan Project, the Apollo Programme, the International Space Station and the development of the GPS system, the fifth most expensive experimental project in history – with an estimated resource use of between €18 billion and €22 billion. And we can say it even better: what the press calls ‘the world’s most complex construction project’, or the largest experimental fission reactor ever built.
We can go even further by explaining, at least succinctly, what fusion is: it is a nuclear reaction in which, when two atoms of deuterium and tritium – isotopes of hydrogen which, over decades of research, have been shown to be the ideal ‘fuel’ – collide, an enormous amount of energy is released, as well as helium atoms and neutrons. We can get an idea of the fusion reaction if we consider that it is the same as the one that produces the energy we get from the Sun.
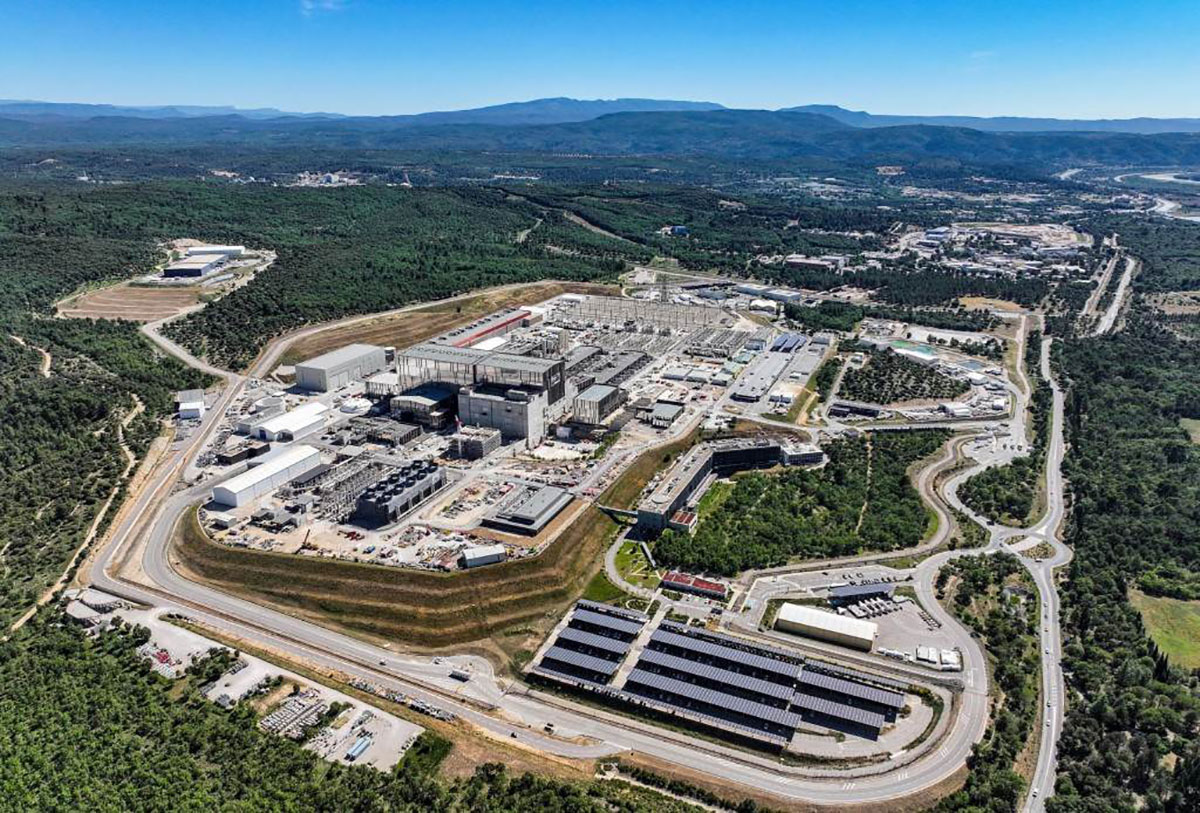
The good thing about this energy source is that it produces no direct radioactive waste and uses virtually inexhaustible fuel. Incidentally, mankind has been pursuing a way to control this immense energy source, in other words, the plasma of deuterium and tritium at millions of degrees Celsius, ever since Australian physicist Mark Oliphant, Austrian chemical physicist Paul Harteck and New Zealand physicist Ernest Rutherford achieved the first fusion reaction at Cambridge University’s Cavendish Laboratories in 1934.
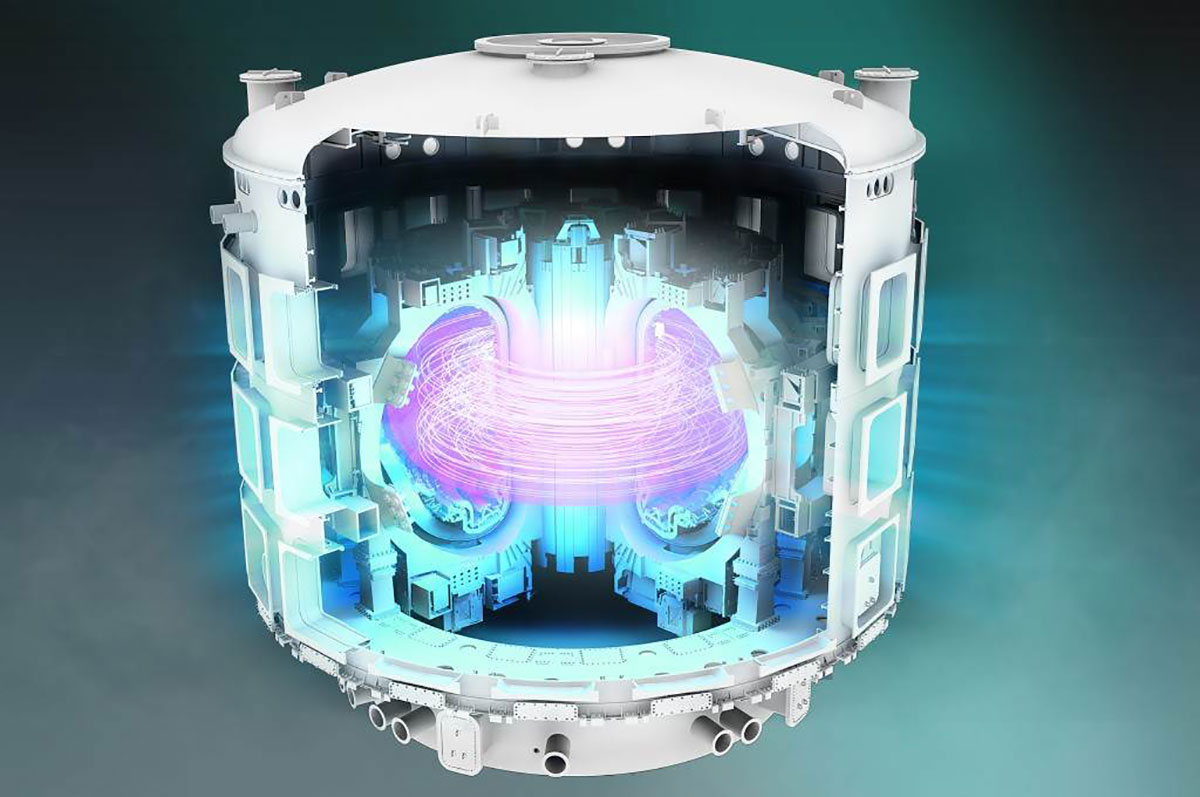
Before going any further, a few words are necessary to explain the whereabouts of the ITER construction: the experiment, which is still under preparation, in other words, under construction, is being carried out in Cadarache, in the south of France. Which leads us to explaining the how; the experimental fission reactor project is being carried out as an international collaboration between Europe, the USA, Russia, China, India, Japan and South Korea.
This scientific collaboration began in 1985 – and here’s when – on the initiative of the Soviet Union’s President Mikhail Gorbachev. After enlisting the support of French President François Mitterrand, Gorbachev proposed the creation of an international project to develop fusion energy for peaceful purposes, to Ronald Reagan, then President of the United States.
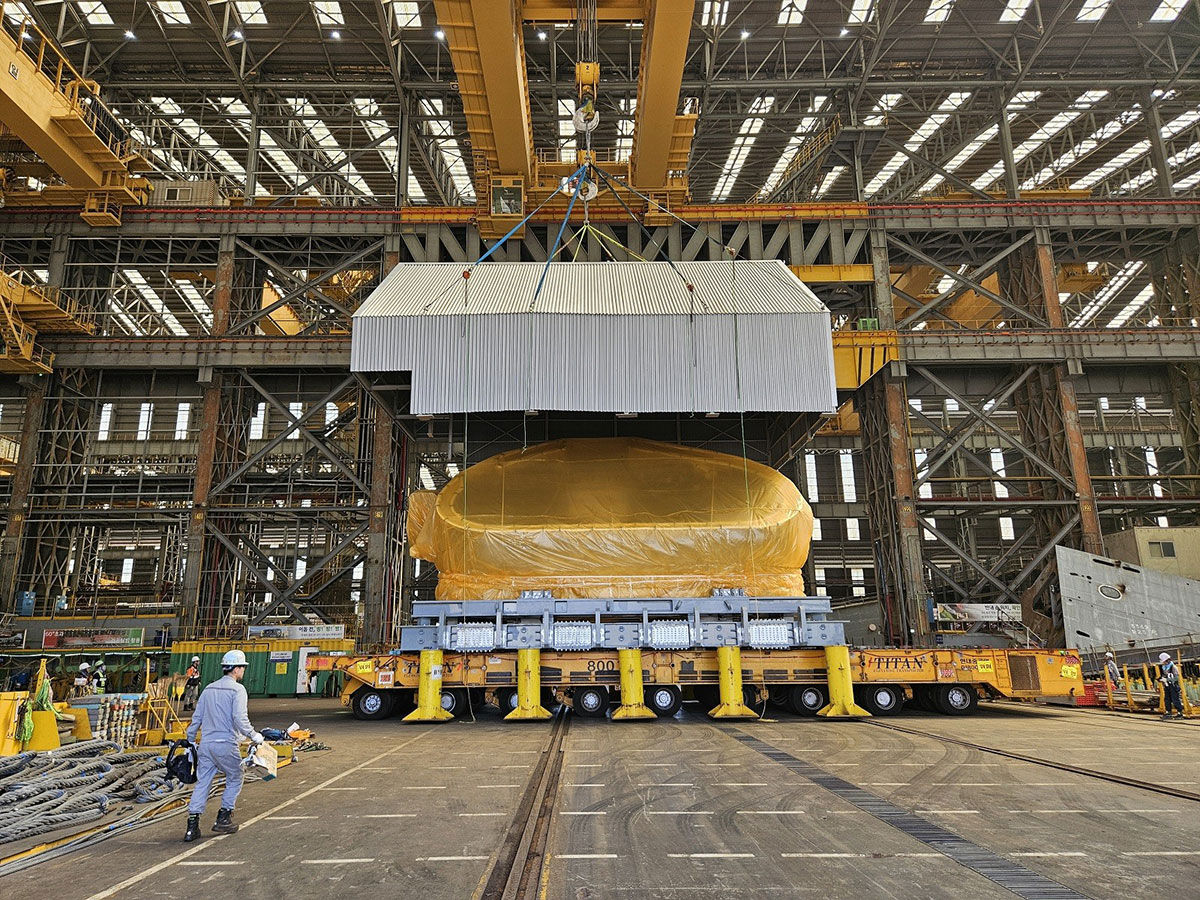
Initially, the agreement was signed by the Soviet Union, the United States, the European Atomic Energy Community (or Euratom, a European public body in charge of coordinating nuclear energy research programmes) and Japan. However, China, South Korea and India joined the project in 2007. Europe, which is funding 50% of ITER, with France contributing 10% and the other partners 10%, has acquired the right to host the site of the experiment, hence its location in Cadarache. Construction is expected to be completed by the end of 2025, with the first deuterium and tritium operations expected in 2035 – and that’s all about the when.
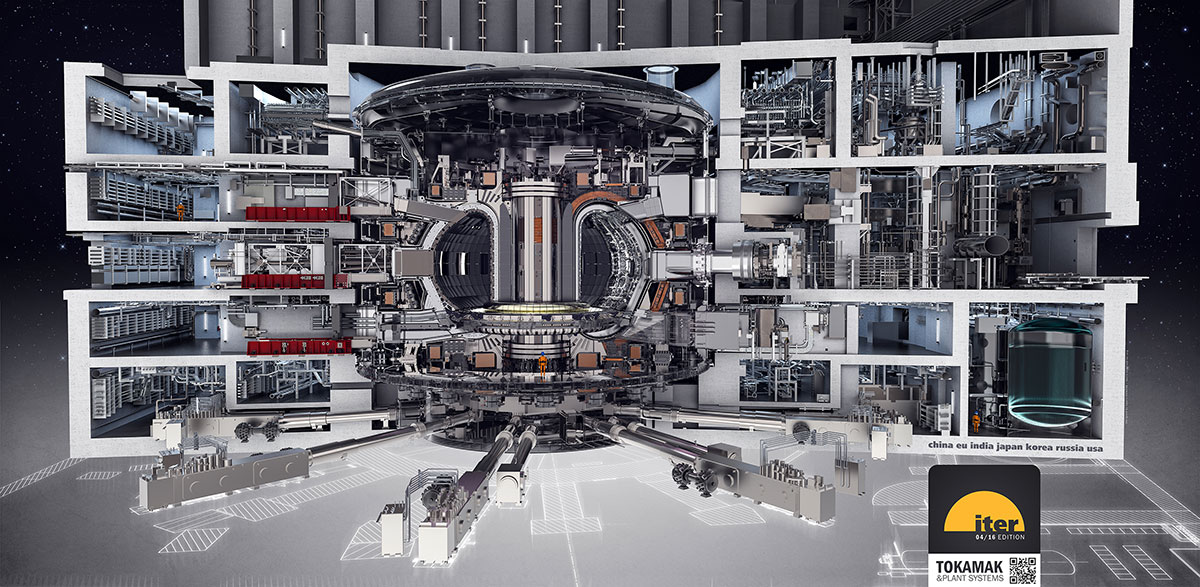
On to the what: 150,000 m3 of concrete, 7,500 tonnes of steel, 1 million components and 10 million individual parts, an estimated 15,000 workers from 5,000 companies in 90 countries – 3,000 people dedicated to civil engineering alone – all contained in 39 buildings and an area of 42 hectares – ‘one of the largest man-made levelled surfaces in the world’. All in all, to build a project that revolves around the tokamak, an acronym from the Russian for Toroidal Magnetic Coil Chamber – the Russians were the first to come up with this design – ‘a ring-shaped magnetic chamber (…), the best fusion reactor design we know of’, Professor Ian Trevelyan Chapman, director general of the UK’s Atomic Energy Authority, told Power Technology.
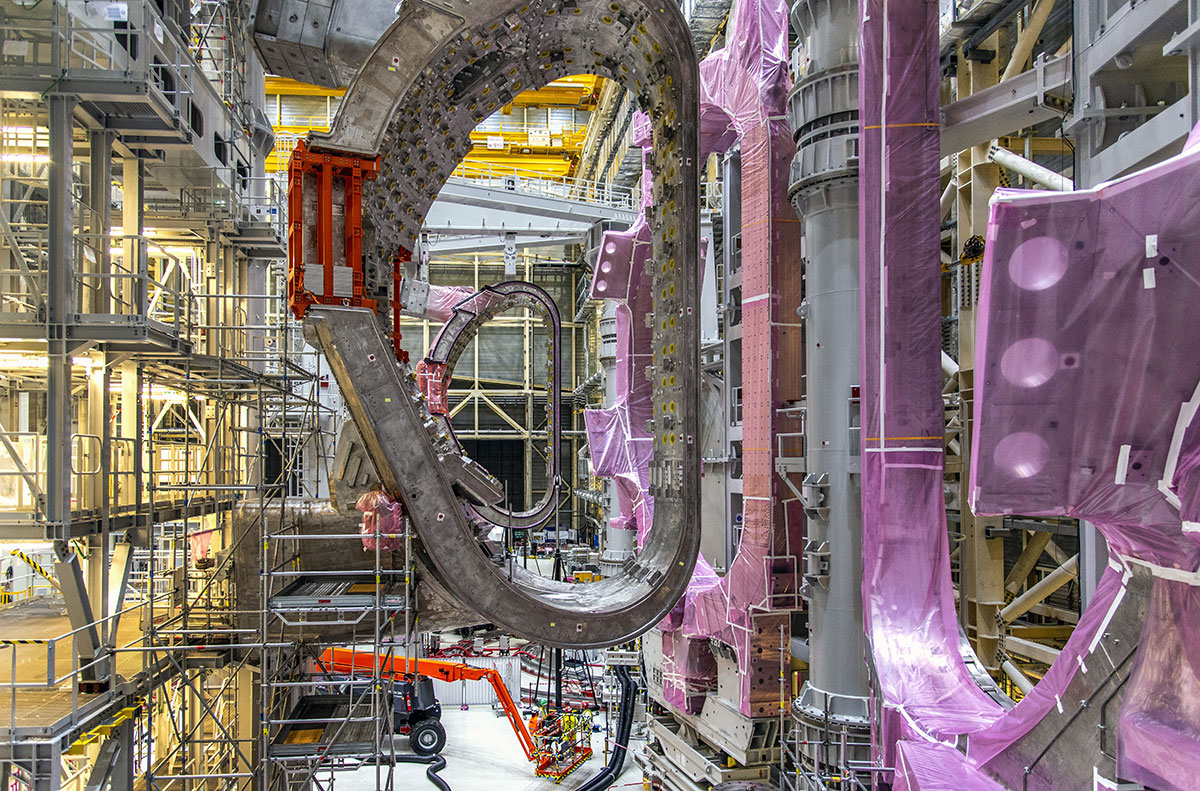
Let’s breathe for a second and move on to what: the construction of a vacuum chamber… no, the world’s largest stainless steel vacuum chamber, weighing 4,000 tonnes, to contain the tokamak; the construction of a system of electromagnets, the most powerful ever built – whose magnetic field will contain the extremely hot plasma of deuterium and tritium – and a system or ‘cryostat’ inside which liquid helium will circulate at temperatures of -269°C – necessary to ensure the superconductivity of the magnets.
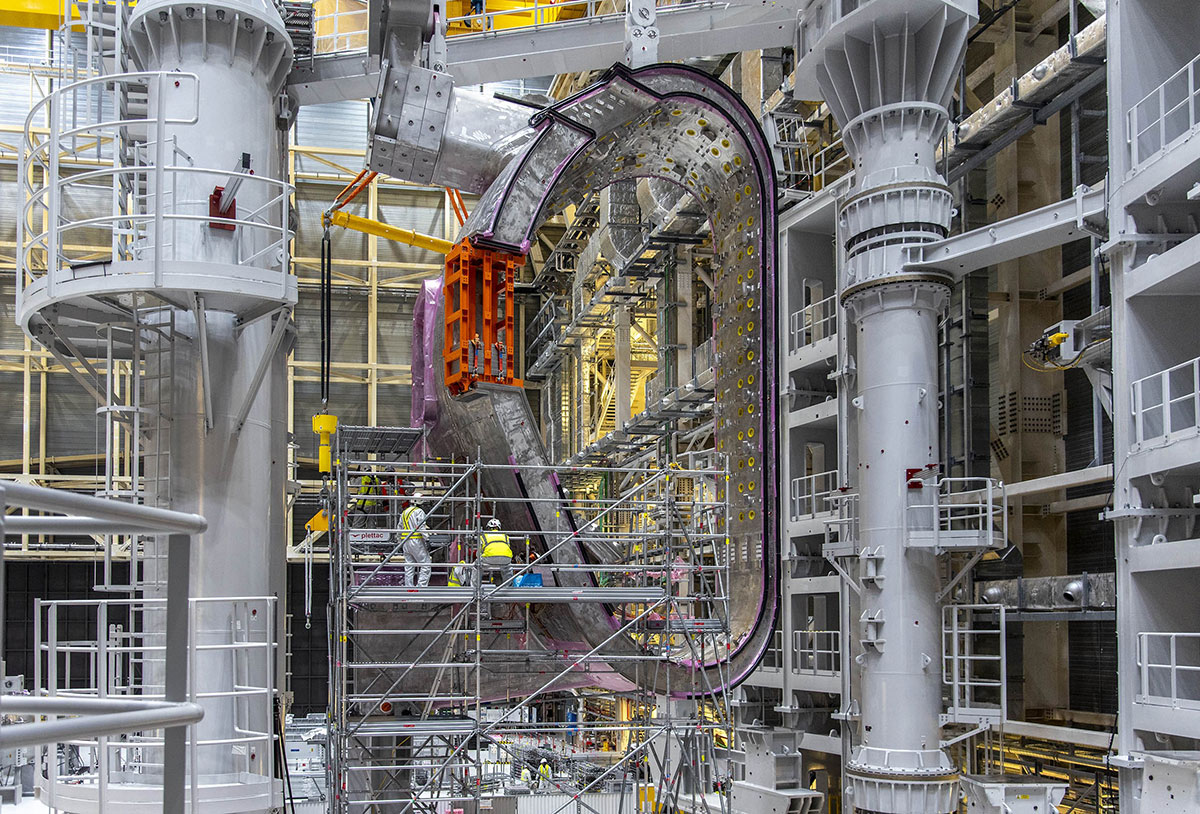
The ultimate goal of all this complex deployment is to reproduce the conditions necessary for the fission reaction to occur so that we can contain and direct it in our favour. That is, to heat the deuterium and tritium fuel to a temperature of 150 million °C so that the atoms of the two substances acquire sufficient velocities to fuse upon collision and that the energy produced is 10 times greater, or 500 MW, than that consumed by the process, in other words, 50 MW.
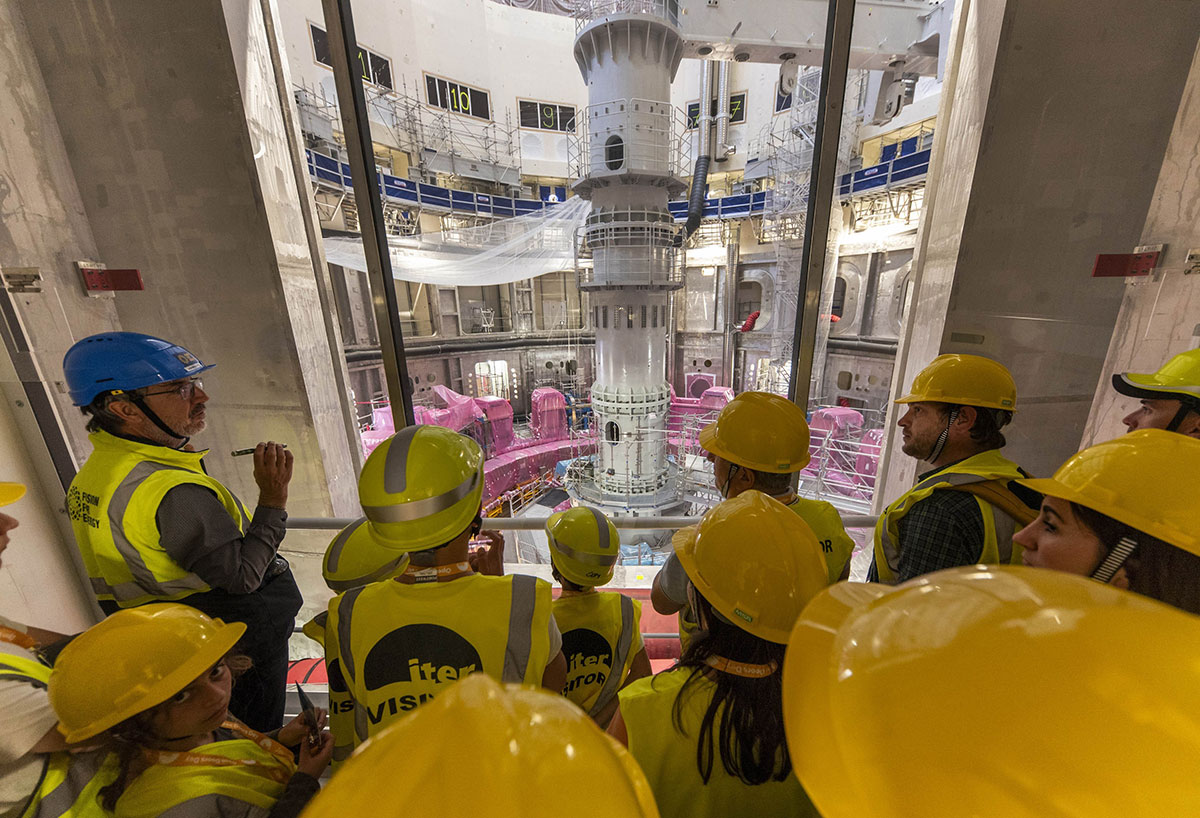
All that remains is to clarify why: because nuclear fusion, if we can control it and put it to work for us, as ITER and many other experiments aim to do, would provide unlimited energy output without CO2 emissions and radioactivity. Or, as Fusion for Energy – the EU organisation managing its contribution to ITER – puts it, ‘to measure its performance, and draw lessons for a future commercial fusion power plant’.
Sources: ITER, Fusion for Energy, The B1M, Power Technology, Wikipedia.
Images: ITER.